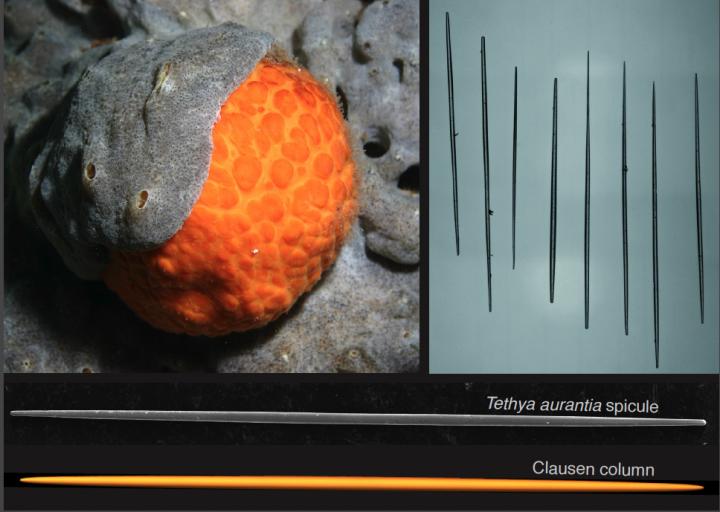
Credit: Michael Monn, Haneesh Kesari / Brown University
PROVIDENCE, R.I. [Brown University] — Judging by their name alone, orange puffball sea sponges might seem unlikely paragons of structural strength. But maintaining their shape at the bottom of the churning ocean is critical to the creatures' survival, and new research shows that tiny structural rods in their bodies have evolved the optimal shape to avoid buckling under pressure.
The rods, called strongyloxea spicules, measure about 2 millimeters long and are thinner than a human hair. Hundreds of them are bundled together, forming stiff rib-like structures inside the orange puffball's spongy body. It was the odd and remarkably consistent shape of each spicule that caught the eye of Brown University engineers Haneesh Kesari and Michael Monn. Each one is symmetrically tapered along its length — going gradually from fatter in the middle to thinner at the ends.
Using structural mechanics models and a bit of digging in obscure mathematics journals, Monn and Kesari showed the peculiar shape of the spicules to be optimal for resistance to buckling, the primary mode of failure for slender structures. This natural shape could provide a blueprint for increasing the buckling resistance in all kinds of slender human-made structures, from building columns to bicycle spokes to arterial stents, the researchers say.
"This is one of the rare examples that we're aware of where a natural structure is not just well-suited for a given function, but actually approaches a theoretical optimum," said Kesari, an assistant professor of engineering at Brown. "There's no engineering analog for this shape — we don't see any columns or other slender structures that are tapered in this way. So in this case, nature has shown us something quite new that we think could be useful in engineering."
The findings are published in the journal Scientific Reports.
Function and form
Orange puffball sponges (Tethya aurantia) are native to the Mediterranean Sea. They live mainly in rocky coastal environments, where they're subject to the constant stress of underwater waves and tidal forces. Sponges are filter feeders — they pump water through their bodies to extract nutrients and oxygen. To do this, their bodies need to be porous and compliant, but they also need enough stiffness to avoid being deformed too much.
"If you compress them too much, you're essentially choking them," Kesari said. "So maintaining their stiffness is critical to their survival."
And that means the spicules, which make up the rib-like structures that give sponges their stiffness, are critical components. When Monn and Kesari saw the shapes of the spicules under a microscope, the consistency of the tapered shape from spicule to spicule was hard to miss.
"We saw the shape and wondered if there might be an engineering principle at work here," Kesari said.
To figure that out, the researchers first needed to understand what forces were acting on each individual spicule. So Monn and Kesari developed a structural mechanics model of spicules bundled within a sponge's ribs. The model showed that the mismatch in stiffness between the bulk of the sponge's soft body and the more rigid spicules causes each spicule to experience primarily one type of mechanical loading — a compression load on each of its ends.
"You can imagine taking a toothpick and trying to squeeze it longways between your fingers," Monn said. "That's how these spicules see the world."
The primary mode of failure for a structure with this mechanical load is through buckling. At a certain critical load, the structure starts to bend somewhere along its length. Once the bending starts, the force transferred by the load is amplified at the bending point, which causes the structure to break or collapse.
Once Kesari and Monn knew what forces were acting on the spicules and how they would fail, the next step was looking to see if there was anything special about them that helped them resist buckling. Scanning electron microscope images of the inside of a spicule and other tests showed that they were monolithic silica — essentially glass.
"We could see that there was no funny business going on with the material properties," Monn said. "If there was anything contributing to its mechanical performance, it would have to be the shape."
Optimal shape
Kesari and Monn combed the literature to see if they could find anything on tapering in slender structures. They came up empty in the modern engineering literature. But they found something interesting published more than 150 years ago by a German scientist named Thomas Clausen.
In 1851, Clausen proposed that columns that are tapered toward their ends should have more buckling resistance than plain cylinders, which had been and still are the primary design for architectural columns. In the 1960s, mathematician Joseph Keller published an ironclad mathematical proof that the Clausen column was indeed optimal for resistance to buckling — having 33 percent better resistance than a cylinder. Even compared to a very similar shape — an ellipse, which is slightly fatter in the middle and pointier at the ends — the Clausen column had 18 percent better buckling resistance.
Knowing what the optimal column shape is, Monn and Kesari started making precise dimensional measurements of dozens of spicules. They showed that their shapes were remarkably consistent and nearly identical to that of the Clausen column.
"The spicules were a match for the best shape of all possible column shapes," Monn said.
It seems in this case, natural selection figured out something that engineers have not. Despite the fact that it's been mathematically shown to be the optimal column shape, the Clausen profile isn't widely known in the engineering community. Kesari and Monn hope this work might bring it out of the shadows.
"We see this as an addition to our library of structural designs," Monn said. "We're not just talking about an improvement of a few percent. This shape is 33 percent better than the cylinder, which is quite an improvement."
In particular, the shape would be particularly useful in a new generation of materials made from nanoscale truss structures. "It would be easy to 3-D print the Clausen profile into these materials, and you'd get a tremendous increase in buckling resistance, which is often how these materials fail."
Lessons from nature
The field of bio-inspired engineering began at a time when many people viewed adaptive evolution as an unceasing march toward perfection. If that were true, scientists should find untold numbers of optimal structures in nature.
But the modern understanding of evolution is a bit different. It's now understood that in order for a trait to be conserved by natural selection, it doesn't need to be optimal. It just needs to be good enough to work. That has put a bit of a damper on the enthusiasm for bio-inspired engineering, Kesari and Monn say.
However, they say, this work shows that nearly optimal structures are out there if researchers look in the right places. In this case, they looked at creatures from a very old phylum — sea sponges are among the very first animals on Earth — with plenty of time to evolve under consistent selection pressures.
Sponges are also fairly simple creatures, so understanding the function of a given trait is relatively straightforward. In this case, the spicule appears to have one and only one job to do — provide stiffness. Compare that to, for example, human bone, which not only provides support but must also accommodate arteries, provide attachment points for muscles and house bone marrow. Those other functions may cause tradeoffs in adaptations for strength or stiffness.
"With the sponges, you have lots of evolutionary pressure, lots of time and opportunity to respond to that pressure, and functional elements that can be easily identified," Kesari said.
With those as guiding principles, there may well be more ideal structures out there waiting to be found.
"This work shows that nature can hit an optimum," Kesari said, "and the biological world can still be hiding completely new designs of considerable technological significance in plain sight."
###
The work was supported by the National Science Foundation (1562656).
Media Contact
Kevin Stacey
[email protected]
401-863-3766
@brownuniversity
http://www.brown.edu/Administration/News_Bureau
############
Story Source: Materials provided by Scienmag