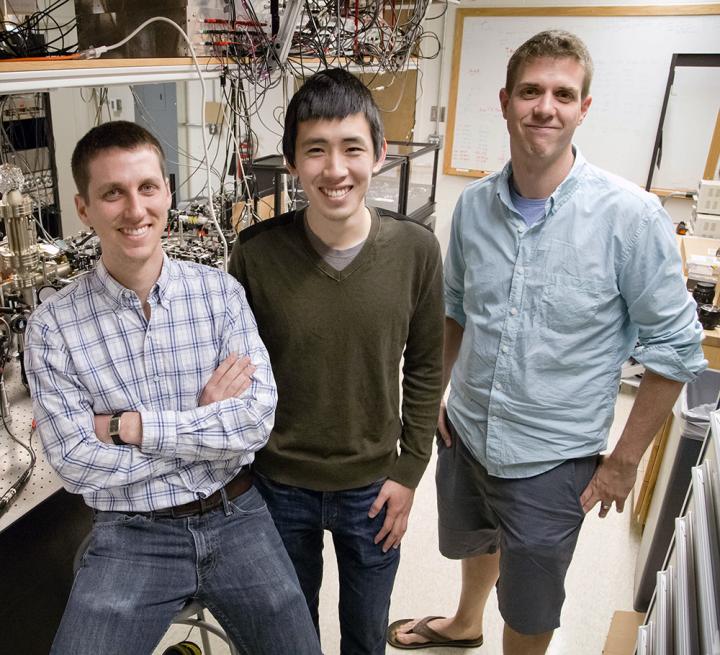
Credit: L. Brian Stauffer, University of Illinois
Topological insulators, an exciting, relatively new class of materials, are capable of carrying electricity along the edge of the surface, while the bulk of the material acts as an electrical insulator. Practical applications for these materials are still mostly a matter of theory, as scientists probe their microscopic properties to better understand the fundamental physics that govern their peculiar behavior.
Using atomic quantum-simulation, an experimental technique involving finely tuned lasers and ultracold atoms about a billion times colder than room temperature, to replicate the properties of a topological insulator, a team of researchers at the University of Illinois at Urbana-Champaign has directly observed for the first time the protected boundary state (the topological soliton state) of the topological insulator trans-polyacetylene. The transport properties of this organic polymer are typical of topological insulators and of the Su-Schrieffer-Heeger (SSH) model.
Physics graduate students Eric Meier and Fangzhao Alex An, working with Assistant Professor Bryce Gadway, developed a new experimental method, an engineered approach that allows the team to probe quantum transport phenomena.
"Quantum simulation allows for some unique capabilities as compared to direct studies of electron transport in real materials," explains Gadway. "A chief advantage of using neutral atoms is the ability to manipulate them at will through the use of laser light and other electromagnetic fields. By changing the details of these control fields, we can, for example, add tailored disorder to study localization phenomena or break symmetries of the system in a controlled way, like through the introduction of a large effective magnetic field. The ultimate goal is to use such a well-controlled system in the regime where particles interact strongly, and explore new phenomena whose emergence we would not have been able to predict based on the behavior of single atoms."
The team's new method takes this idea of system design, or "Hamiltonian engineering," to the extreme, allowing the researchers to control every single element that governs the transport of single particles.
"This particular study was important because we showed for the first time that we can use this method to realize topologically nontrivial systems, and there's strong promise for the future realization of interacting, topological systems of atoms." Meier comments. "Ours is the first study of this kind to allow site-resolved detection of the topological boundary states and the probing of their structure in a phase-sensitive way." The results are published in the December 23, 2016 issue of Nature Communications.
The Su-Schrieffer-Heeger model is the textbook model of a topological insulator, displaying most of the salient features associated with topological systems–a topological phase with protected boundary states and an insulating system bulk. In conjugated polymers like polyacetylene, the topological soliton state is associated with the dimerized structure of alternating single and double bonds along the molecule's backbone chain. Protected electronic states show up at the boundary between regions with opposite alternating order, and give rise to some unique transport properties, including an increase in electrical conductivity by about nine orders of magnitude under light doping with impurities.
An explains, "Some of the most interesting aspects of topological systems are rather subtle or rely on fine-tuning of the system parameters. Engineered quantum systems–cold atoms, photonic simulators, superconducting qubits, etc.–are better equipped for the exploration of these types of phenomena. The reason for this is that they're generally free from the intrinsic disorder, both material disorder and thermal fluctuations, that would be hard to avoid in a conventional condensed matter system."
The team's new technique holds promise for further investigations into the fundamental behavior of topological systems. Additional experiments are already underway, extending this work to two-dimensional quantum Hall-type systems and the exploration of topological insulators in the presence of disorder.
"The interesting aspect of our study is that we were able to observe directly the topological boundary states of this system and probe them in a phase-sensitive way with atomic physics techniques," Gadway sums up. "Future experiments, similar in vein but in a slightly different experimental system, could allow for the exploration of strongly correlated transport phenomena inaccessible by classical simulation. The biggest goal of our group in the near future is observing the influence of atomic interactions in such a system. In particular, the fact that our atoms form an interacting quantum fluid allows them to naturally support local interactions in the engineered model system. We're hoping to probe the influence of these interactions very soon."
###
Media Contact
Bryce Gadway
[email protected]
217-244-7559
@EngineeringAtIL
http://engineering.illinois.edu/
############
Story Source: Materials provided by Scienmag