A protein from bovine blood can keep gold nanoparticles from clumping in a solution—a discovery that could lead to improved biomedical applications.
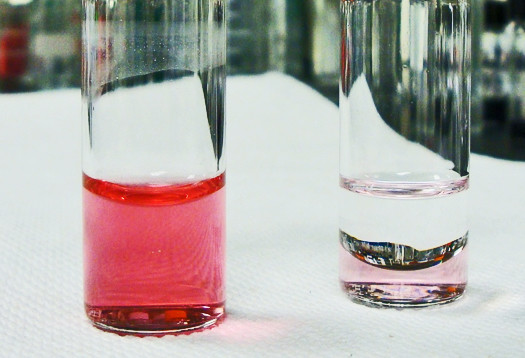
Bovine serum albumin (BSA) forms a protein “corona” around gold nanoparticles that keeps them from aggregating, particularly in high-salt environments like seawater.
The new research from the labs of Rice University chemists Stephan Link and Christy Landes could also assist in project that use nanoparticles in harsh environments. The findings appears in the journal ACS Sustainable Chemistry and Engineering.
Link’s primary interest is in the plasmonic properties of nanoparticles. Landes’ work incorporates protein binding and molecular transport. The BSA research combines their unique talents with those of Sergio Dominguez-Medina, a graduate student in Link’s lab.
“Initially, we wanted to look at nanoparticles in solution with something they would encounter frequently in blood: serum albumin,” Landes says. “In our first experiments, Sergio reported the very efficient, reasonably fast and irreversible binding the moment he put nanoparticles into a solution that contained serum albumin.”
“It turned out the salt is actually driving this binding,” Dominguez-Medina says.
Without BSA, gold nanoparticles in a salty solution quickly aggregate and fall to the bottom. “That by itself is undesirable for biomedical or industrial applications, because it could lead to toxicity issues,” he says.
“The nanoparticles get more hydrophobic because in the presence of salts, the excess charges on the surface (which discourage clumping) are actually removed.” But if BSA is present, the proteins are drawn to the nanoparticles faster than the particles are drawn to each other.
“Once the protein is bound, it gives a super protection against any type of salt-induced aggregation. We think this could be used for the stabilization of nanoparticles in environments where, right now, it hasn’t been achieved,” Dominguez-Medina says.
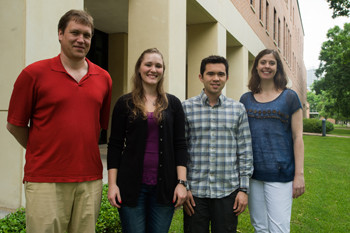
He says the discovery also offers the possibility that nanoparticles might be made more compatible for treating humans by using a patient’s own albumin. “Albumin is really easy to purify and the process is well-established,” he says.
Protecting surfaces
The ability of gold nanoparticles to absorb and redirect light is at the heart of several breakthrough technologies being developed. Most notable are a nanoparticle-based cancer treatment now in human testing that was developed by Professor Naomi Halas and former colleague Jennifer West, and Halas’ project to convert solar energy directly into steam for sanitation and water purification.
“The only way nanoparticles exhibit their really nice optical properties in very specific optical frequencies is if they’re separated,” Landes says.
Because pure gold nanoparticles are so hydrophobic, they naturally clump together in a solution unless chemically treated. “A lot of industrial effort goes into keeping stuff off of surfaces, like contact lenses and ship hulls,” she says. “That involves chemically altering the surfaces to prevent unwanted adsorption, or in the case of nanoparticles, unwanted aggregation.”
Protecting the surface is costly, Link says. “But we found we could take nanoparticles prepared in the cheapest way, with a sodium citrate coating that stabilizes the particles by electrostatic repulsion, and add BSA, which coats the particles and makes them really stable.”
Albumin is the most common protein in blood, and the bovine version shares 98 percent of its amino acid sequence with human serum albumin. “One of its main purposes, biologically, is to take things that aren’t water-soluble, bind to them and make them soluble,” Landes says. “When you combine it with gold nanoparticles, BSA trades places with the cheap citrate, which isn’t a good protective layer, to form the monolayer corona, which is very strong and protective.”
Seawater is the very definition of a harsh environment, Landes says. “One of the problems with desalination applications and, similarly, with fuel cells, is that saline or acidic conditions are very corrosive,” she says. “That’s why you have to use platinum electrodes in fuel cells—not because they’re better than cheaper materials at catalysis, but because they don’t corrode in a harsh environment.” She sees promise for BSA-treated gold nanoparticles in both applications.
Nanorods next
The researchers are now looking at how well gold nanoparticles retain their albumin corona with repeated use. “Gold is expensive,” Landes says. “But the beauty of it is that if you can reuse it, it only costs you once.”
They also want to use spectroscopy to see how the binding mechanism works in real time, Link says. “We want to study what’s happening at the interface of nanoparticles and biologically relevant media” that may eventually include DNA, RNA, and drugs for delivery to cells, he says.
Link plans to see how BSA can be used in combination with gold nanorods. Because nanorods’ plasmonic properties can be tuned, “we can get them into the biological window, which is near-infrared light,” he says. Near-IR from lasers is used to activate, by heating, Halas’ and West’s cancer-killing nanoshells. Nanorods may also offer ways to combine BSA and other useful proteins by coating the tips and sides separately.
Co-authors are Jan Blankenburg, an exchange student at Rice from the University of Mainz in Germany, and Jana Olson, a graduate student and National Science Foundation (NSF) fellow in Link’s lab.
The Robert A. Welch Foundation, the Cancer Prevention and Research Institute of Texas, the Army Research Office, the NSF, and National Institutes of Health supported the research.
Story Source:
The above story is reprinted from materials provided by Rice University, Mike Williams.